- 1Department of Clinical Science, Bergen Integrated Diagnostic Stewardship Cluster, Faculty of Medicine, University of Bergen, Bergen, Norway
- 2Department of Microbiology, Haukeland University Hospital, University of Bergen, Bergen, Norway
- 3Department of Infectious Diseases, Oslo University Hospital, Oslo, Norway
- 4National Institute of Public Health, University of Southern Denmark, Copenhagen, Denmark
- 5Department of Physiology, St. John’s Medical College, Bangalore, India
- 6Division of Health and Humanities, St. John’s Research Institute, Bangalore, India
- 7GlaxoSmithKline Vaccines, Wavre, Belgium
Delays in diagnosis and treatment of pulmonary tuberculosis (TB) can lead to more severe disease and increased transmission. Contact investigation among household contacts (HHCs) of TB patients is crucial to ensure optimal outcomes. In the context of a prospective cohort study in Palamaner, Southern India, this study attempted to assess the potential of 27 different soluble immune markers to accurately assign HHCs for appropriate treatment. A multiplex bead assay was applied on QuantiFERON (QFT)-nil supernatants collected from 89 HHCs grouped by longitudinal QFT status; M. tuberculosis (Mtb) infected (QFT positive at baseline and follow-up, n = 30), recent QFT converters (QFT-negative at baseline, n = 27) and converted to QFT-positivity within 6 months of exposure (at follow-up, n = 24) and QFT consistent negatives (n = 32). The 29 TB index cases represented Active TB. Active TB cases and HHCs with Mtb infection produced significantly different levels of both pro-inflammatory (IFNγ, IL17, IL8, IP10, MIP-1α, MIP1β, and VEGF) and anti-inflammatory (IL9 and IL1RA) cytokines. We identified a 4-protein signature (bFGF, IFNγ, IL9, and IP10) that correctly classified HHCs with Mtb infection vs. Active TB with a specificity of 92.6%, suggesting that this 4-protein signature has the potential to assign HHCs for either full-length TB treatment or preventive TB treatment. We further identified a 4-protein signature (bFGF, GCSF, IFNγ, and IL1RA) that differentiated HHCs with Mtb infection from QFT consistent negatives with a specificity of 62.5%, but not satisfactory to safely assign HHCs to no preventive TB treatment. QFT conversion, reflecting new Mtb infection, induced an elevated median concentration in nearly two-thirds (19/27) of the analyzed soluble markers compared to the levels measured at baseline. Validation in other studies is warranted in order to establish the potential of the immune biosignatures for optimized TB case detection and assignment to therapeutic and preventive treatment of Mtb infected individuals.
Introduction
Even before the impact of the COVID-19 pandemic on health system capacity, the decline in TB incidence by 2019 was already too slow to reach the first milestone of the End TB Strategy, with still an estimated number of 10.0 million new TB cases annually (1). The COVID-19 pandemic has impacted everyday life, damaged economies, and overwhelmed health care systems (2, 3). The interruption of health services to a point where identification of new TB cases and treatment initiation decreased by 25–50% over 3 months (between April and June, 2020) may upsurge TB deaths by 0.2–0.4 million globally in 2020 alone (1). Notably, early identification and treatment is a priority of the End TB Strategy to minimize transmission mandatory to reach the goal of eliminating the TB epidemic (4, 5).
The risk of TB progression is highest in newly exposed subjects; thus, active case finding in the households of newly diagnosed TB index patients is considered an effective strategy (6). The purpose of contact investigations is to identify subjects with increased risk of TB progression in order to offer preventive TB treatment (7). The WHO recommendations for preventive TB treatment depend on the national health resource setting and the epidemiological context. In low- and middle-income countries, preventive TB treatment is recommended for high-risk subjects such as children aged below 5 years and people living with HIV or other immunosuppressant conditions. Active TB must be ruled out by assuring the absence of the hallmark TB symptoms; cough, fever, weight loss and night sweats (8). In order to achieve early case identification and avoid TB progression with the risk of subsequent transmission, TB control programs could profit from more ambitious goals: To identify all household contacts with active/subclinical TB or M. tuberculosis (Mtb) infection at risk of TB progression, and, dependent on the condition, assign appropriate treatment. For active/subclinical TB (drug-sensitive Mtb isolates) this would be a full 6-month course (2-month intensive phase; isoniazid, rifampicin, pyrazinamide, and ethambutol) followed by a 4-month continuation phase; isoniazid, rifampicin), and for Mtb infected a prophylactic course of 3 months (isoniazid, rifampicin/rifapentine) either once-weekly or daily, or 9 months (isoniazid) either twice-weekly or daily (9). Accurate assignment of patients is vital to avoid under- and over-treatment, risking either relapse and/or Mtb resistance in the case of undertreating, or side-effects without gain in the case of overtreating (10).
It has repeatedly been shown that despite heavy and prolonged Mtb exposure, a certain proportion of subjects does not develop detectable T cell responses (for e.g., they remain QFT negative through repeated testing). These subjects have the lowest risk of TB progression, suggesting that they possess effective natural TB immunity, likely mediated by innate immune mechanisms (11, 12). No proxy marker or biosignature for this kind of natural protective immunity has been established, but finding such a biomarker or biosignature is considered the holy grail within TB immunology (13): Immunobiomarkers in TB may be useful to understand the role of host responses in natural protection or vulnerability towards Mtb infection, but also hold a potential for new diagnostic tools and proxy-readouts for vaccine and/or treatment efficacy (14).
In the setting of a longitudinal study of index TB cases and their household contacts, the Household Contact Cohort (HHC) study, conducted in Palamaner, Southern India, in the period 2010–2012, aimed to explore the potential of 27 soluble immune markers detected in QuantiFERON (QFT) nil tube supernatants to accurately assign TB-exposed subjects within a household to either i) standard TB treatment (concomitant active and/or subclinical TB cases) or ii) preventive treatment (Mtb infected with risk of TB progression) (15) or eventually iii) no treatment in subjects that likely possess natural immunity. Furthermore, we aimed to explore if the selected markers could provide some insight into natural TB immunity.
Methods and Materials
Ethical Consideration
Ethical approval for this study was obtained from the Institutional Ethical Review Board (IERB) of St. John’s Medical College, Bangalore (IERB/1/527/08). The material transfer agreement between St. John’s Medical College, Bangalore, and the University of Bergen, Norway, was obtained from the Department of Biotechnology, Government of India (No. BT/Med.II/Adv (SS)/Misc./02/2012). Ethical approval was also obtained from Western Norway’s Regional Committee for Medical and Health Research Ethics (Ref no: 2018/1614 D).
Study Population
The present study is a cross-sectional study nested within a prospective cohort study of adult PTB index cases and their household contacts and conducted at our study site at the Emmaus Swiss Leprosy Project and Referral Hospital, Palamaner and Kuppam Taluks, Chittoor district, Andhra Pradesh, India (3.200°N, 72.7500°E, altitude 683 m) between September 2010 and April 2012. In total, 176 pulmonary TB (PTB) index cases were identified at the microscopy centers of the Revised National Tuberculosis Control Program (RNTCP) run by the Government of India and 164 recruited after written informed consent. In the context of our study, TB was confirmed in 150 cases by the presence of Mtb in sputum smear and/or culture. Positive cultures were confirmed by using the GenoType MTBC kit (Hain Life science GmBH, Nehren, Germany). Direct PCR by COBAS Taqman MTB test (Roche Diagnostics Ltd, Rotkreuz, Switzerland) was undertaken on culture-negative specimen for patients with chest X-ray (CXR) finding suggestive of TB. Recruited PTB index cases were treated with standard anti-TB treatment (ATT) and followed until completion of the 6-month treatment course. A total of 525 household contacts of the 176 PTB index cases were recruited. Household contacts who were not part of the 150 Mtb culture-positive index TB cases were also included for the investigation. Written informed consent was provided by all adults. For children ≤7 years, parents/guardians provided written informed consent, and for participants >7 years, an additional written assent was obtained. The intended follow-up for all household contacts was one year.
Clinical Assessments and Sampling
Baseline assessments of PTB index cases and household contacts: Medical history (namely, BCG vaccination status, history of TB exposure, prior TB/ATT, socio-economic status, and risk factors such as diabetes, smoking, and alcohol consumption), socio-demographic, anthropometric, and clinical data were recorded. At baseline, a tuberculin skin test (TST) was performed by a trained nurse (2 TU/0.1 ml tuberculin; Span Diagnostics, Surat, India) and read after 48–72 h; an induration >10 mm was defined as positive. Blood 3 ml was collected for the QuantiFERON-TB Gold In-tube (QFT-GIT) assay and the supernatants (plasma) from the Nil, TB antigen (ag) and Mitogen tubes were collected in two microfuge tubes and stored at −80°C. One microfuge tube was used for the QFT-GIT assay and the other tube was stored for Bio-Plex assay. Three independent radiologists interpreted the CXR (anteroposterior view) at baseline, and agreement by at least two was required for the assignment abnormal TB chest X-ray. CXR was again done at the end of the study closure for all participants. Since the prevalence of HIV in India is low [<0.5%] (16), after pre-test counseling, only participants who volunteered for HIV testing were tested. Agreement for HIV testing was not a criterion for inclusion.
Sample Selection and Definition of Groups
PTB index cases were selected to represent Active TB disease (Group 1) as concomitant cases in the household were referred to the RNTCP and excluded from the HHC. Stored blood samples available from 109 of 150 Mtb culture-positive participants, and only 48 of 109 blood samples were obtained before ATT initiation for QFT, of which only 39 index TB cases had either previously diagnosed TB >3 years or no prior TB reported. These 39 samples were considered to include in this study. However, QFT Nil tube samples were available for 29 of 39 PTB index cases and constituted Group 1, Active TB disease.
Household contacts being QFT positive at baseline and at repeated testing at 2-, 6-, and 12-month follow-up and did not develop TB disease, were assigned as QFT consistent positive Mtb infected (Group 2) and were considered representative of historical and/or likely recent Mtb exposure.
Household contacts, who were QFT negative at baseline but converted to QFT positivity at 2- or 6-month follow-up and did not develop TB disease, were assigned to recent QFT converters (Group 3) and were considered representative of recent Mtb exposure.
Household contacts that remained QFT negative throughout the 12-month follow-up and that did not develop TB were assigned to QFT consistent negatives (Group 4) and were considered representative of likely natural protective innate mechanisms towards TB.
Both Mtb infected (QFT consistent positive) and recent QFT converters (QFT positivity at 2- or 6-month follow-up) were considered representative of contacts that would profit from preventive treatment without risk of undertreating subclinical TB/active TB. Therefore, these two groups were merged and called Household contacts with Mtb infection.
Sample selection is shown in Figure 1. For the purpose of this study, Active TB (n = 29), QFT consistent positive Mtb infected (n = 30; hereafter referred to as Mtb infected throughout this manuscript), recent QFT converters (n = 27 who were QFT-negative at baseline but who converted to QFT-positivity within 6 months of exposure; 14 converted at 2 months and 10 converted at 6 months and for 3 samples the follow-up Nil-ag tubes were not available) and QFT consistent negatives (n = 32). In total, 142 samples were included for the biomarker analysis.
Multiplex Cytokine/Chemokine Assays
For the Bio-Plex Multiplex Immunoassays, we used the Nil tube supernatants from the QFT-GIT assay. This unstimulated control sample can be used as a substitute for separate serum stored samples (17). The selected 142 samples were tested using the ‘Human cytokines 27-plex’ kit (Bio-Rad Laboratories Inc., Hercules, CA, USA), according to the instructions of the manufacturer. The 27 biomarkers included are Interleukin (IL)-1β, IL-1 receptor antagonist (IL1-ra), IL-2, IL-4, IL-5, IL-6, IL-7, IL-8/CXCL8, IL-9, IL-10, IL-12 (p70), IL-13, IL-15, IL-17, eotaxin/CXCL11, basic fibroblast growth factor (bFGF), granulocyte-colony stimulating factor (G-CSF), granulocyte–macrophage colony-stimulating factor (GM-CSF), interferon (IFN)-γ, interferon-inducible protein (IP-10)/CXCL10, monocyte chemotactic protein (MCP-1)/CCL2, macrophage inflammatory protein (MIP)-1α/CCL3, MIP-1β/CCL4, platelet-derived growth factor-BB (PDGF-BB), regulated upon activation T cell expressed and secreted (RANTES)/CCL5, tumor necrosis factor (TNF), and vascular endothelial growth factor (VEGF). Data acquisition was performed on a Luminex100 analyzer (Luminex Corporation, Austin, Texas, USA) according to the instructions of the manufacturer. Cytokine/Chemokine concentrations were measured in (pg ml−1).
Data Analysis
Patient characteristics were summarized as mean (standard deviation), median (interquartile range; IQR), and counts, as appropriate. Between-group comparisons of biomarker levels across participant groups (Active TB (Group 1), Mtb infected (Group 2), recent QFT converters (Group 3), and QFT consistent negatives (Group 4) were carried out applying the Kruskal–Wallis using Dunn’s post-hoc test. Within-group comparisons of biomarker levels between baseline and follow-up samples carried out applying Wilcoxon matched paired test.
Analyses aiming to identify diagnostic biosignatures for which a two-step approach was applied (18). In brief, we first applied logistic regression selecting biomarkers for the Lasso regression analysis. A predicted probability >0.5 resulted in classification as participants with Mtb infection/disease and <0.5 as participants without Mtb infection/disease. The sensitivity and specificity for the identified signatures were defined by their ability to assign a correct high probability (above 0.5) to participants as either TB cases or controls. The diagnostic abilities of the signatures were summarized by means of receiver operator characteristic (ROC) curves (area under the curve [AUC], sensitivity, and specificity). Analyses were carried out using R (R Core Team, 2019) (19) through the interface RStudio (www.rstudio.com).
Results
Characterization of the Study Participants at Baseline
A total of 118 study participants were classified into four groups; Active TB (n = 29), Mtb infected (n = 30), recent QFT converters (baseline n = 27; follow-up n = 24), and QFT consistent negatives (n = 32). The mean age was 45.6 years (SD± 13.9) for Active TB, 22.0 years (SD± 15.3) for Mtb infected, 29.5 years (SD± 19.8) for recent QFT converters, and 18.3 years (SD± 16.9) for QFT consistent negatives. Males constituted 79.3% (23/29) of Active TB, 36.6% (11/30) of Mtb infected, 48.1% (13/27) of recent QFT converters, and 50.0% (16/32) of QFT consistent negatives. As expected, the groups with negative QFT at baseline (QFT converters and QFT consistent negatives) all had a TST <10 mm. Therefore, the only meaningful comparison was between Active TB (median TST 15 mm) and Mtb infected (median TST 14.5 mm). Interestingly, but consistent with reduced QFT sensitivity in active TB, median IFNγ concentration was higher in Mtb infected compared to Active TB. Complete clinical characteristics are shown in Table 1.
Cytokines Patterns in Active TB Cases and Exposed Household Contacts at Baseline
Twenty-seven different cytokines, including chemokines and markers of inflammation, were analyzed in QFT-GIT Nil tube supernatants, and compared between Active TB cases and household contacts grouped by Mtb infection status following exposure as described. Compared to Active TB, Mtb infected exhibited higher levels of the inflammatory T-cell cytokines IFNγ, IL9, and IL17, and the vascular endothelial growth factor (VEGF), whereas the pro-inflammatory IP10, released from innate cells in response to IFNγ was higher in Active TB (Figure 2).
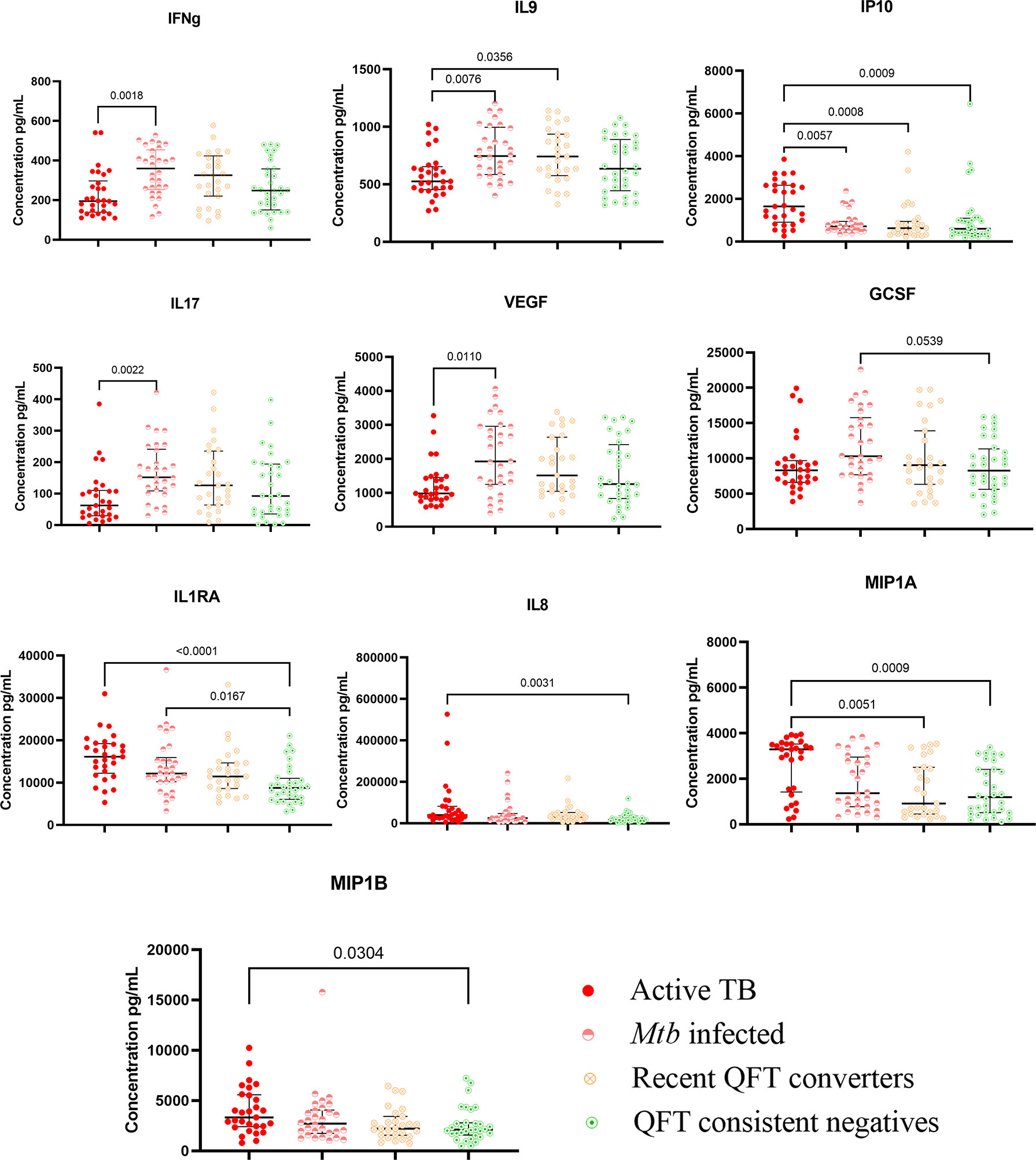
Figure 2 Kruskal–Wallis test with Dunn’s post correction was applied. Scatter-plot graph depicting median cytokine concentrations (pg ml−1) from the QFT-GIT supernatants (nil tube) by the Multiplex bio-plex assay. Nil levels (pg ml−1) of IFNγ, IL9, IP10, IL-17, VEGF, GCSF, IL1RA, IL8, MIP1α, and MIP1β in patients with active TB (ATB; n = 29), Mtb infected (n = 30), recent QFT converters (n = 27), and QFT consistent negatives (n = 32).
Compared to Active TB, QFT consistent negatives had lower levels of the innate pro-inflammatory IL8 promoting neutrophil chemotaxis, macrophage inflammatory protein (MIP)-1α and -1β and IP10, and the anti-inflammatory interleukin 1 receptor antagonist (IL1RA) that inhibits IL-1 effects. Compared to Mtb infected, QFT consistent negatives had lower levels of the innate pro-inflammatory cytokine granulocyte colony-stimulating factor (GCSF). QFT consistent negatives also exhibited lower levels of the anti-inflammatory IL1RA compared to Active TB and Mtb infected (Figure 2).
Dynamics in Cytokine Patterns in Recent QFT Converters
Compared to baseline, the concentrations of 19 out of 27 cytokine/chemokine markers were significantly elevated in recent QFT converters at conversion that occurred within 6 months of continuous exposure to index TB cases (Figure 3). All these 19 cytokine/chemokine markers play a role in the development of inflammatory and protective immune responses to microbial invaders by modulating immune cells of both the innate and adaptive immune systems. Notably, all the 19 markers were elevated in the recent QFT converter follow-up samples as compared to the other groups (Supplementary Table 1).
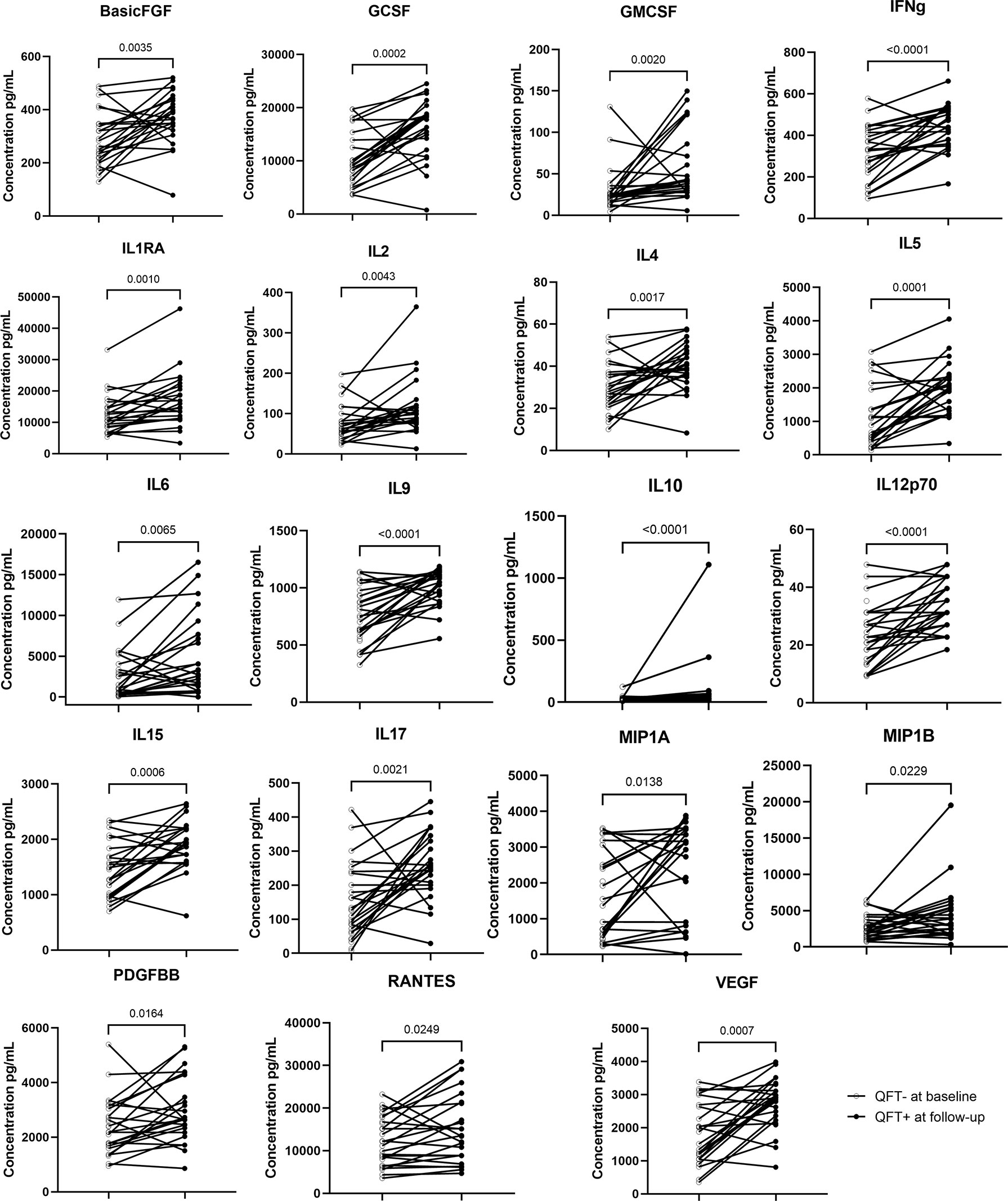
Figure 3 Wilcoxon matched paired test was applied. Symbols and lines graph depicting individual changes in cytokines/chemokines concentrations (pg ml−1) from recent QFT converters in baseline samples (n = 27) to recent QFT converters in the follow-up sample (n = 24).
Protein Signatures With a Potential to Discriminate Active TB From Mtb Infected and Recent QFT Converters
To identify a protein signature associated with Active TB, univariate feature selection was applied using logistic regression (18), and identified a 6-protein signature, comprising bFGF, GCSF, IFNγ, IL9, IP10, and MIP1α (Table 2A) that differentiated Active TB from Mtb infected with an AUC of 0.89 (95% CI, 0.81–0.98; Figure 4A), sensitivity of 79.3% (95% CI, 60.3–92.0) and specificity of 83.3% (95% CI, 65.3–98.9) (Table 2B). Similarly, a 5-protein signature was identified, comprising IFNγ, IL1RA, IL9, IP10, and MIP1α that differentiated Active TB from recent QFT converters with an AUC of 0.87 (95% CI, 0.76–0.96; Figure 4B), sensitivity of 75.9% (95% CI, 56.5–89.7) and specificity of 81.5% (95% CI, 61.9–94.4). Interestingly, 4 proteins (IFNγ, IL9, IP10, and MIP1α) overlapped between the two identified signatures, suggesting differential expression of these is associated with active TB disease, rather than infection per se.
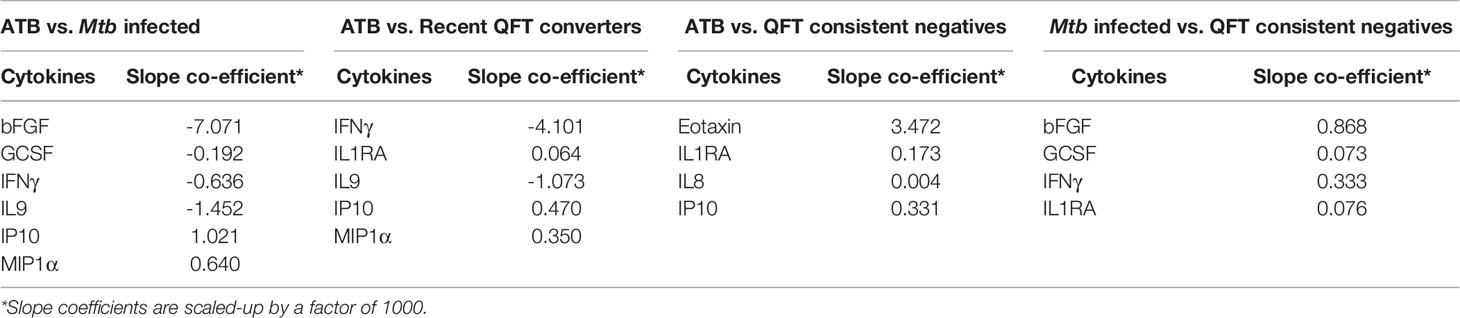
Table 2a Expression and regression coefficients for each biomarker of the identified protein signature.
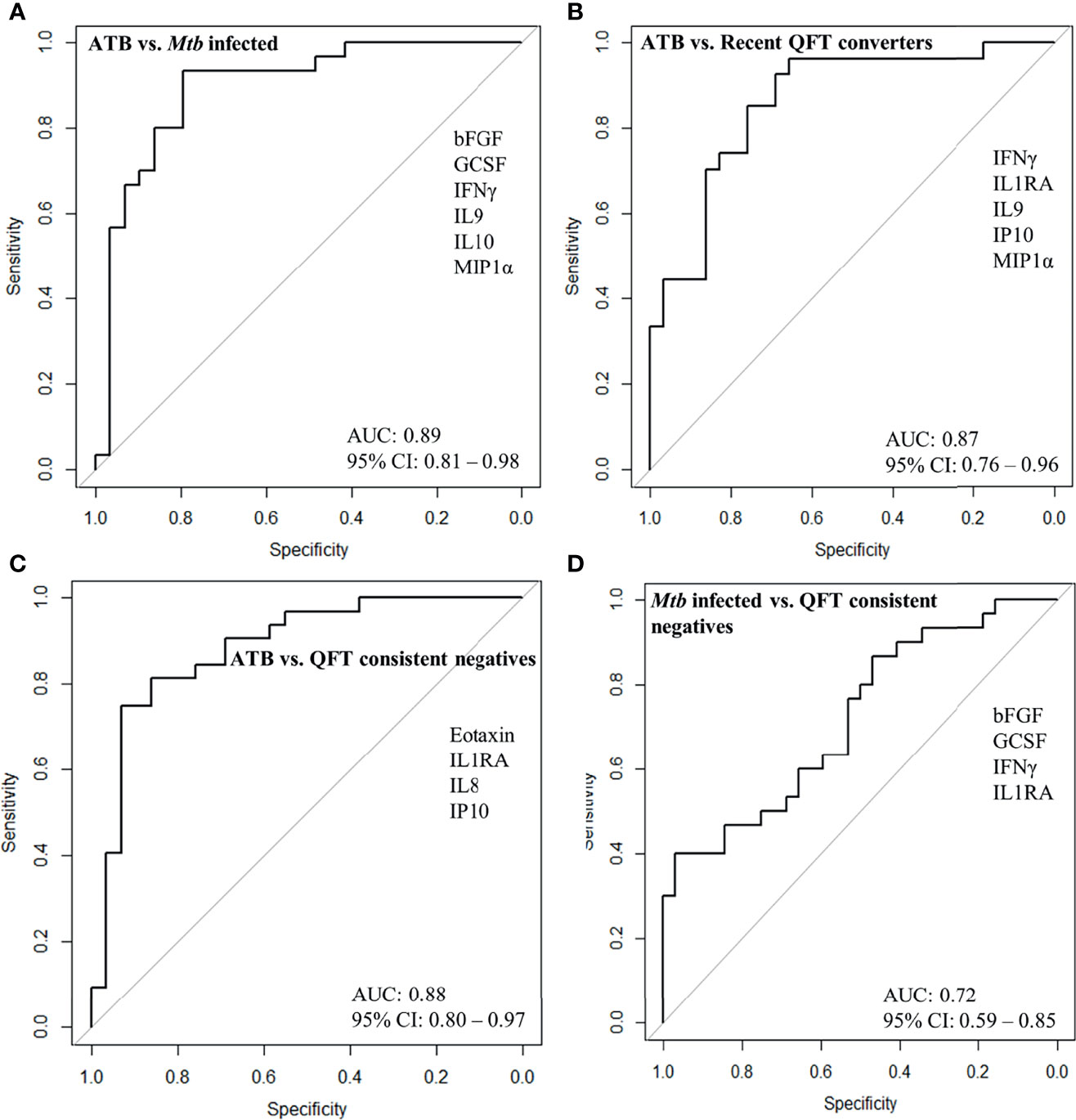
Figure 4 ROC curves for protein signature that distinguishes (A) Active TB (ATB; n = 29) from Mtb infected (n = 30), (B) ATB (n = 29) from recent QFT converters (n = 27), (C) ATB (n = 29) from QFT consistent negatives (n = 32), and (D) Mtb infected (n = 30) from QFT consistent negatives (n = 32).
Accuracy of Protein Signatures to Assign Therapeutic or Prophylactic TB Treatment
Next, we merged household contacts that could profit from preventive TB treatment, namely Mtb infected, sampled at baseline, and recent QFT converters, sampled at the time of/after QFT conversion. This group, called Household contacts with Mtb infection (merged Mtb infected + recent QFT converters), was then compared against Active TB to identify a protein signature. Univariate feature selection was followed by lasso regression analysis. A 4-protein signature was identified, comprising bFGF, IFNγ, IL9, and IP10 (Table 3A) that differentiated Active TB from Household contacts with Mtb infection with an AUC of 0.89 (95% CI, 0.80–0.96; Figure 5A), sensitivity of 72.4% (95% CI, 52.8–87.3) and specificity of 92.6% (95% CI, 82.1–97.9) (Table 3B).

Table 3a Expression and regression coefficients for each biomarker of the identified protein signature.
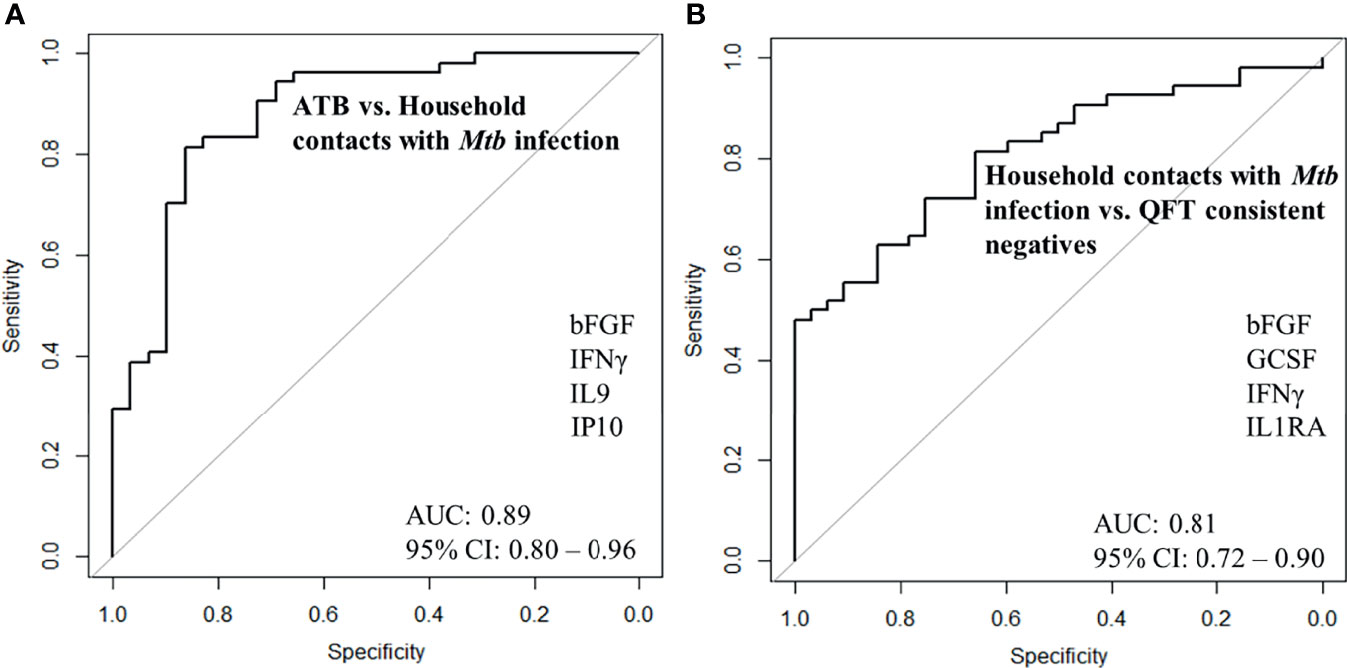
Figure 5 ROC curves for signature that distinguishes (A) Active TB (n = 29) from Household contacts with Mtb infection (n = 30), (B) Household contacts with Mtb infection (n = 30) from QFT consistent negatives (n = 32).
Protein Signatures Distinguishing QFT Consistent Negatives From Active TB and Mtb Infected
QFT consistent negatives were satisfactorily discriminated from Active TB by a 4-protein signature, comprising Eotaxin, IL1RA, IL8, and IP10 that differentiated with an AUC of 0.88 (95% CI, 0.80–0.97; Figure 4C), sensitivity of 86.2% (95% CI, 68.3–96.1), and specificity of 81.3% (95% CI, 63.6–92.8). In addition, QFT consistent negatives could to some extent be discriminated from Mtb infected by another 4-protein signature comprising bFGF, GCSF, IFNγ, and IL1RA (Table 2A) with an AUC of 0.72 (95% CI, 0.59–0.85; Figure 4D), sensitivity of 50.0% (95% CI, 31.3–68.7), and specificity of 71.9% (95% CI, 53.3–86.3) (Table 2B). Notably, IL1RA overlapped between the two identified signatures.
Accuracy of Protein Signatures to Safely Assign no TB Treatment
Next, Household contacts with Mtb infection (merged Mtb infected + recent QFT converters) were compared to QFT consistent negatives by lasso analysis. A 4-protein signature was identified, comprising bFGF, GCSF, IFNγ, and IL1RA (Table 3A) that differentiated Household contacts with Mtb infection from QFT consistent negatives with an AUC of 0.81 (95% CI, 0.72–0.90; Figure 5B), sensitivity of 87.0% (95% CI, 75.1–94.6), and specificity of 62.5% (95% CI, 43.7–78.9; Table 3B) for safe assignment to no TB treatment.
Discussion
The natural history of TB is characterized by a dynamic spectrum of disease, in which newly infected individuals are at the highest risk of developing active disease, but may also enter a state of long-term latent infection from which they may progress to disease many years after infection (20). Understanding where infected individuals are in this spectrum from infection to disease progression is the key to appropriate management and treatment. The purpose of contact investigations following identification of a TB index case is early case detection to limit morbidity and further transmission. This can be achieved by i) identification of concomitant TB cases in need of a therapeutic course of TB treatment, and ii) identification of individuals with recently acquired Mtb infection and subsequent elevated risk of TB progression who would benefit from preventive therapy or attentive clinical surveillance (21). We identified a 6-protein signature (bFGF, GCSF, IFNγ, IL9, IP10, and MIP1α) that distinguished Active TB from Mtb infected, and a 4-protein signature (Eotaxin, IL1RA, IL8, and IP10) that distinguished Active TB from QFT consistent negatives with AUCs of 0.89 and 0.88, respectively.
In order to guide the assignment of HHCs to full-length TB treatment (Active TB) or preventive TB treatment (Mtb infected), we compared Active TB vs. Household contacts with Mtb infection (merged Mtb infected + recent QFT converters) and identified a 4-protein signature (bFGF, IFNγ, IL9, and IP10) with a specificity of 92.6%. Even with an accurate biomarker signature to identify concomitant TB cases in a household, we acknowledge the need for respiratory samples and microbiological verification before initiation of TB treatment to ascertain the diagnosis and drug sensitivity. Nevertheless, a screening test for Mtb infection could accurately rule out concomitant TB and limit the number of HHCs in need for the sampling for a respiratory specimen and positively assigning HHCs for preventive TB treatment with low risk of undertreating concomitant incipient and subclinical TB cases. Hopefully, the screening test would also identify and secure appropriate treatment of extrapulmonary TB cases for which microbiological verification is far more challenging. Further, to identify HHCs for whom treatment could safely be withheld due to likely natural TB immunity, we compared QFT consistent negatives with Household contacts with Mtb infection and identified a 4-protein signature (bFGF, GCSF, IFNγ, and IL1RA) that correctly classified QFT consistent negatives with an AUC of 0.81 and a disappointing specificity of 62.5%. Interestingly, bFGF and IFNγ overlapped between the two identified signatures. In TB pathology, T cells produce IFNγ, which aids in protective immunity against M. tuberculosis by activating macrophages, allowing them to eliminate Mtb more effectively (22). Still, although essential for anti-mycobacterial responses, IFNγ alone is not sufficient, and high levels of IFNγ-producing T cells do not correlate with immune protection (23). bFGF is also associated with tissue regeneration and angiogenesis, but we observed reduced levels in Active TB compared to HHCs with Mtb infection (QFT consistent positives).
The innate immune response that controls Mtb infection starts with pathogen recognition and uptake by resident tolerant alveolar macrophages in which Mtb finds a niche for replication. Once the bacterial load reaches beyond “the tolerant threshold”, local early innate responses are activated and start producing cytokines, chemokines, and antimicrobial molecules resulting in bacterial killing/restricted growth and initiation of adaptive immune responses (24). The relevance of exploring the biomarker potential of cytokines and chemokines, and the importance of the IFNγ cytokine in TB immunology has already been mentioned. Regarding chemokines, these are essential for the recruitment of the first line of innate immune effector cells to infection and inflammatory sites and the deployment of natural immune sentinels at mucosal barriers (25). Further, the plasma levels of chemokines have the capacity to influence host immunity to sustain the protective immune response (26). Another likely advantage is that many chemokines, namely, MCP-1, MIP1α, MIP-1β, and IP-10, in adults have been reported probably not to associate with malnutrition, a major risk factor for TB globally (27), whereas this is more uncertain with TST and QFT results. Studies have reported that IP10 is a potential biomarker for TB and contributes to restricting Mtb replication in host tissues (28–32). Interestingly, mRNA levels of the cytokines CCL4 (MIP1β), CXCL10 (IP10) have been reported to decrease following isoniazid preventive treatment (33), suggesting a broad potential for chemokine biomarker tools in diagnosis and prediction of progression and evaluation of treatment response (34–36).
In our study, QFT conversion was indeed reflected in alterations in the analyzed soluble markers as nearly two-thirds (19/27) had increased median concentration from baseline to the time of/after QFT conversion (recent QFT converters). This provides insight in the early chemokines/cytokines-driven inflammatory response induced by Mtb infected/activated macrophages that result in recruitment and activation of innate and adaptive immune cells to the lung (26, 37). In response to granulocyte–macrophage colony-stimulating factor, monocytes, neutrophils, epithelial cells, and adipocytes produce IL-1RA (38), the level of IL1RA was elevated in Active TB in this study. A study has reported that the level of IL-1RA was higher in patients with delayed treatment response than those with a favorable response to therapy (39). IL-9 is mainly produced by Th2 cells, promotes the pleural mesothelial cell repairing, and inhibits IFNγ-induced pleural mesothelial cell apoptosis (33). This is also consistent with a host protective response that aims to prevent excessive pathology (40). IL-9 is involved in the immune-pathogenesis of inflammatory diseases and maintaining immune tolerance, putting it in a similar role to the IL1RA. In TB, IL-17 has been suggested to protect against disease progression and pathological damage in the lung by initial neutrophil recruitment after Mtb infection (41). As reported in a previous study (42), the levels of IL-9 and IL-17 (measured in QFT-Nil tube plasma samples) were reduced in active TB compared to Mtb infection. We report that the pro-inflammatory IP10 was higher in Active TB compared to the other groups, and this finding is consistent with previous studies conducted in various populations (34, 42, 43). IP-10 also plays a chemotactic role, though it seems to be more critical for T cells. The elevated expression of these cytokines in active TB is associated with the infiltration and consolidation seen in the lungs of TB patients and suggests the elevated expression of these genes acts as a marker for TB pathology rather than Mtb infection (38). In the present study, the levels of chemokines IL-8, MIP-1α and MIP-1β and were elevated in active TB, IL8 and MIP-1α attract both lymphocytes and polymorphonuclear neutrophils. IL-8 is involved in the recruitment of leukocytes and the formation of granulomas. Further, MIP-1α from neutrophils may contribute to the recruitment of mononuclear cells after neutrophils have accumulated. Therefore, the production of IL-8 and MIP-1α may be greater in suppurative inflammation than in mycobacterial inflammation (39, 44).
The study reported here has some limitations, principally the fact that it analyzed a limited number of cytokines and chemokines. Moreover, the household contacts were younger than the TB index cases and there was an uneven sex distribution. Immune responses changes with age and this may have had an impact on host protein levels and the identified protein signatures. Further, the risk of TB-exposure per se, but also for repeated TB exposure, increases with age. By design, eligible TB index cases were adults, thereby inducing an inevitable age bias in the Active TB group. A sub-analysis undertaken in the QFT consistent negatives (n = 32), whom can be assumed to be unaffected by the probable age-dependant increase in TB exposure, did not find any significant correlation between age and cytokine concentrations. In addition, no formal sample size calculation was carried out since the maximum sample size was limited by the availability of samples for biomarker analysis and the inability to cross-validate the identified proteomic signature due to the lack of comparable samples from other cohorts. In this study, TB treatment was started in index TB cases based on recommendations, which included the presence of clinical symptoms and also microbiological and/or radiological findings. Even with a valid biomarker signature to identify concomitant TB cases in a household, we acknowledge the need for respiratory samples and microbiological verification before initiation of TB treatment to ascertain the diagnosis and drug sensitivity. Nevertheless, based on these results, further exploration of biosignatures and validation of suggested signatures in broad settings, is warranted in order to establish their potential and eventual use to guide optimal TB case detection and assignment to therapeutic and preventive treatment to Mtb infected individuals. Optimal individual management is currently our best option to change TB epidemiology.
Data Availability Statement
The original contributions presented in the study are included in the article/Supplementary Material. Further inquiries can be directed to the corresponding authors.
Ethics Statement
Ethical approval for this study was obtained from the Institutional Ethical Review Board (IERB) of St. John’s Medical College, Bangalore (IERB/1/527/08). The material transfer agreement between St. John’s Medical College, Bangalore, and the University of Bergen, Norway, was obtained from the Department of Biotechnology, Government of India (No. BT/Med.II/Adv (SS)/Misc./02/2012). Ethical approval was also obtained (Ref no: 2018/1614 D) from Western Norway’s Regional Committee for Medical and Health Research Ethics. Written informed consent to participate in this study was provided by the participants’ legal guardian/next of kin.
Author Contributions
DS, SJ, TMD, CR, and HMSG conceptualized and designed the biomarker study. MV coordinated patient recruitment and follow-up. DS and SJ wrote the manuscript with contributions from TMD, CR, and HMSG. DS performed all laboratory experiments, data analysis and generated the tables and figures. CR supervised the statistical analysis, wrote the section on statistical analysis, and reviewed the manuscript. HMSG had primary responsibility for the final content of the manuscript. All authors listed have made a substantial, direct, and intellectual contribution to the work and approved it for publication.
Funding
The study is supported by the Research Council of Norway Global Health and Vaccination Research (GLOBVAC) projects: RCN 179342, 192534, and 248042, the University of Bergen (Norway); the St. John’s Research Institute, Bangalore. Helse-vest grant (F-10441).
Conflict of Interest
TMD is an employee of and holds shares in the GSK group of companies but participated in the current work as an independent investigator.
The remaining authors declare that the research was conducted in the absence of any commercial or financial relationships that could be construed as a potential conflict of interest.
Publisher’s Note
All claims expressed in this article are solely those of the authors and do not necessarily represent those of their affiliated organizations, or those of the publisher, the editors and the reviewers. Any product that may be evaluated in this article, or claim that may be made by its manufacturer, is not guaranteed or endorsed by the publisher.
Acknowledgments
We thank Drs. Rajini Macaden, Nelson Jesuraj, the late John Kenneth, Anto Jesuraj Udaykumar, and Vandana A at St. John’s Research Institute, Bangalore. We thank Aeras (a non-profit organization; now a part of IAVI), USA for their contributions to establishing the TB vaccine trial site at Palamaner Taluk, Chittoor district, Andhra Pradesh, India.
Supplementary Material
The Supplementary Material for this article can be found online at: https://www.frontiersin.org/articles/10.3389/fimmu.2022.801616/full#supplementary-material
References
1. World Health Organization. Global Tuberculosis Report (2020). Available at: https://apps.who.int/iris/bitstream/handle/10665/336069/9789240013131-eng.pdf (Accessed on Oct 25, 2021).
2. Visca D, Ong CWM, Tiberi S, Centis R, D'Ambrosio L, Chen B, et al. Tuberculosis and COVID-19 Interaction: A Review of Biological, Clinical and Public Health Effects. Pulmonology (2021) 27(2):151–65. doi: 10.1016/j.pulmoe.2020.12.012
3. Migliori GB, Tiberi S, Garcia-Basteiro AL, Duarte R. Tuberculosis and its Future in the COVID-19 Era: The Pulmonology Series 2021. Pulmonology (2021) 27(2):94–6. doi: 10.1016/j.pulmoe.2020.10.005
4. World Health Organization. Recommendations for Investigating Contacts of Persons With Infectious Tuberculosis in Low- and Middle-Income Countries (2012). Available at: http://www.who.int/tb/areas-of-work/laboratory/contact-investigation/en/.pdf (Accessed on Oct 25, 2021).
5. World Health Organization. Systematic Screening for Active Tuberculosis: Principles and Recommendations (2013). Available at: http://www.who.int/tb/publications/tbscreening/en/.pdf (Accessed on Oct 25, 2021).
6. Chawla S, Gupta V, Gour N, Grover K, Goel PK, Kaushal P, et al. Active Case Finding of Tuberculosis Among Household Contacts of Newly Diagnosed Tuberculosis Patients: A Community-Based Study From Southern Haryana. J Family Med Prim Care (2020) 9(7):3701–6. doi: 10.4103/jfmpc.jfmpc_532_20
7. World Health Organization. Latent Tuberculosis Infection: Updated and Consolidated Guidelines for Programmatic Management (2018). Available at: https://apps.who.int/iris/bitstream/handle/10665/260233/9789241550239-eng.pdf (Accessed on Oct 25, 2021).
8. World Health Organization. WHO Consolidated Guidelines on Tuberculosis Module 1: Prevention Tuberculosis Preventive Treatment (2020). Available at: https://apps.who.int/iris/bitstream/handle/10665/331170/9789240001503-eng.pdf (Accessed on Oct 25, 2021).
9. Sterling TR, Njie G, Zenner D, Cohn DL, Reves R, Ahmed A, et al. Guidelines for the Treatment of Latent Tuberculosis Infection: Recommendations From the National Tuberculosis Controllers Association and CDC, 2020. MMWR Recomm Rep (2020) 69(1):1–11. doi: 10.15585/mmwr.rr6901a1
10. Diallo T, Adjobimey M, Ruslami R, Trajman A, Sow O, Obeng Baah J, et al. Safety and Side Effects of Rifampin Versus Isoniazid in Children. N Engl J Med (2018) 379(5):454–63. doi: 10.1056/NEJMoa1714284
11. Kaipilyawar V, Salgame P. Infection Resisters: Targets of New Research for Uncovering Natural Protective Immunity Against Mycobacterium Tuberculosis. F1000Res (2019) 8:F1000 Faculty Rev-1698.. doi: 10.12688/f1000research.19805.1
12. Foster M, Hill PC, Setiabudiawan TP, Koeken V, Alisjahbana B, van Crevel R. BCG-Induced Protection Against Mycobacterium Tuberculosis Infection: Evidence, Mechanisms, and Implications for Next-Generation Vaccines. Immunol Rev (2021) 301(1):122–44. doi: 10.1111/imr.12965
13. Scriba TJ, Netea MG, Ginsberg AM. Key Recent Advances in TB Vaccine Development and Understanding of Protective Immune Responses Against Mycobacterium Tuberculosis. Semin Immunol (2020) 50:101431. doi: 10.1016/j.smim.2020.101431
14. Maitra A, Kamil TK, Shaik M, Danquah CA, Chrzastek A, Bhakta S. Early Diagnosis and Effective Treatment Regimens are the Keys to Tackle Antimicrobial Resistance in Tuberculosis (TB): A Report From Euroscicon's International TB Summit 2016. Virulence (2017) 8(6):1005–24. doi: 10.1080/21505594.2016.1256536
15. Drain PK, Bajema KL, Dowdy D, Dheda K, Naidoo K, Schumacher SG, et al. Incipient and Subclinical Tuberculosis: A Clinical Review of Early Stages and Progression of Infection. Clin Microbiol Rev (2018) 31(4):e00021–18. doi: 10.1128/CMR.00021-18
16. Bachani D, Sogarwal R. National Response to HIV/AIDS in India. Indian J Community Med (2010) 35(4):469–72. doi: 10.4103/0970-0218.74341
17. Kimuda SG, Andia-Biraro I, Egesa M, Bagaya BS, Raynes JG, Levin J, et al. Use of QuantiFERON(R)-TB Gold in-Tube Culture Supernatants for Measurement of Antibody Responses. PloS One (2017) 12(11):e0188396. doi: 10.1371/journal.pone.0188396
18. Gjoen JE, Jenum S, Sivakumaran D, Mukherjee A, Macaden R, Kabra SK, et al. Novel Transcriptional Signatures for Sputum-Independent Diagnostics of Tuberculosis in Children. Sci Rep (2017) 7(1):5839. doi: 10.1038/s41598-017-05057-x
19. R Core Team. A Language and Environment for Statistical Computing. Vienna, Austria: R Foundation for Statistical Computing (2019). Available at: https://www.r-project.org (Accessed Oct 25, 2021).
20. Havumaki J, Cohen T, Zhai C, Miller JC, Guikema SD, Eisenberg MC, et al. Protective Impacts of Household-Based Tuberculosis Contact Tracing are Robust Across Endemic Incidence Levels and Community Contact Patterns. PloS Comput Biol (2021) 17(2):e1008713. doi: 10.1371/journal.pcbi.1008713
21. European Centre for Disease Prevention and Control. Management of Contacts of MDR TB and XDR TB Patients (2012). Available at: https://www.ecdc.europa.eu/sites/portal/files/media/en/publications/Publications/201203-Guidance-MDR-TB-contacts.pdf (Accessed on Oct 25, 2021).
22. Sharma S, Bose M. Role of Cytokines in Immune Response to Pulmonary Tuberculosis. Asian Pac J Allergy Immunol (2001) 19(3):213–9.
23. Huang L, Russell DG. Protective Immunity Against Tuberculosis: What Does it Look Like and How do We Find it? Curr Opin Immunol (2017) 48:44–50. doi: 10.1016/j.coi.2017.08.001
24. Orme IM, Robinson RT, Cooper AM. The Balance Between Protective and Pathogenic Immune Responses in the TB-Infected Lung. Nat Immunol (2015) 16(1):57–63. doi: 10.1038/ni.3048
25. Sokol CL, Luster AD. The Chemokine System in Innate Immunity. Cold Spring Harb Perspect Biol (2015) 7(5):a016303. doi: 10.1101/cshperspect.a016303
26. Monin L, Khader SA. Chemokines in Tuberculosis: The Good, the Bad and the Ugly. Semin Immunol (2014) 26(6):552–8. doi: 10.1016/j.smim.2014.09.004
27. Kathamuthu GR, Sridhar R, Baskaran D, Babu S. Low Body Mass Index has Minimal Impact on Plasma Levels of Cytokines and Chemokines in Tuberculous Lymphadenitis. J Clin Tuberc Other Mycobact Dis (2020) 20:100163. doi: 10.1016/j.jctube.2020.100163
28. Joosten SA, van Meijgaarden KE, Arend SM, Prins C, Oftung F, Korsvold GE, et al. Mycobacterial Growth Inhibition is Associated With Trained Innate Immunity. J Clin Invest (2018) 128(5):1837–51. doi: 10.1172/JCI97508
29. Delemarre EM, van Hoorn L, Bossink AWJ, Drylewicz J, Joosten SA, Ottenhoff THM, et al. Serum Biomarker Profile Including CCL1, CXCL10, VEGF, and Adenosine Deaminase Activity Distinguishes Active From Remotely Acquired Latent Tuberculosis. Front Immunol (2021) 12:725447. doi: 10.3389/fimmu.2021.725447
30. Palucci I, Battah B, Salustri A, De Maio F, Petrone L, Ciccosanti F, et al. IP-10 Contributes to the Inhibition of Mycobacterial Growth in an Ex Vivo Whole Blood Assay. Int J Med Microbiol (2019) 309(5):299–306. doi: 10.1016/j.ijmm.2019.05.005
31. Santos VS, Goletti D, Kontogianni K, Adams ER, Molina-Moya B, Dominguez J, et al. Acute Phase Proteins and IP-10 as Triage Tests for the Diagnosis of Tuberculosis: Systematic Review and Meta-Analysis. Clin Microbiol Infect (2019) 25(2):169–77. doi: 10.1016/j.cmi.2018.07.017
32. Petrone L, Vanini V, Chiacchio T, Petruccioli E, Cuzzi G, Schinina V, et al. Evaluation of IP-10 in Quantiferon-Plus as Biomarker for the Diagnosis of Latent Tuberculosis Infection. Tuberculosis (Edinb) (2018) 111:147–53. doi: 10.1016/j.tube.2018.06.005
33. de Oyarzabal E, Garcia-Garcia L, Rangel-Escareno C, Ferreyra-Reyes L, Orozco L, Herrera MT, et al. Expression of USP18 and IL2RA Is Increased in Individuals Receiving Latent Tuberculosis Treatment With Isoniazid. J Immunol Res (2019) 2019:1297131. doi: 10.1155/2019/1297131
34. Chegou NN, Black GF, Kidd M, van Helden PD, Walzl G. Host Markers in QuantiFERON Supernatants Differentiate Active TB From Latent TB Infection: Preliminary Report. BMC Pulm Med (2009) 9:21. doi: 10.1186/1471-2466-9-21
35. Jacobs R, Malherbe S, Loxton AG, Stanley K, van der Spuy G, Walzl G, et al. Identification of Novel Host Biomarkers in Plasma as Candidates for the Immunodiagnosis of Tuberculosis Disease and Monitoring of Tuberculosis Treatment Response. Oncotarget (2016) 7(36):57581–92. doi: 10.18632/oncotarget.11420
36. Chendi BH, Snyders CI, Tonby K, Jenum S, Kidd M, Walzl G, et al. A Plasma 5-Marker Host Biosignature Identifies Tuberculosis in High and Low Endemic Countries. Front Immunol (2021) 12:608846. doi: 10.3389/fimmu.2021.608846
37. Guirado E, Schlesinger LS, Kaplan G. Macrophages in Tuberculosis: Friend or Foe. Semin Immunopathol (2013) 35(5):563–83. doi: 10.1007/s00281-013-0388-2
38. Chegou NN, Heyckendorf J, Walzl G, Lange C, Ruhwald M. Beyond the IFN-Gamma Horizon: Biomarkers for Immunodiagnosis of Infection With Mycobacterium Tuberculosis. Eur Respir J (2014) 43(5):1472–86. doi: 10.1183/09031936.00151413
39. Lee JH, Chang JH. Changes of Plasma Interleukin-1 Receptor Antagonist, Interleukin-8 and Other Serologic Markers During Chemotherapy in Patients With Active Pulmonary Tuberculosis. Korean J Intern Med (2003) 18(3):138–45. doi: 10.3904/kjim.2003.18.3.138
40. Alvarez I, Rovetta A, Hernández Del Pino R, Pasquinelli V, Peña D, Musella R, et al. IL-9-Producing Cells Promote Th1 Responses Against Mycobacterium Tuberculosis. (P3015). J Immunol (2013) 190(1 Supplement):55.8–.8.
41. Cruz A, Fraga AG, Fountain JJ, Rangel-Moreno J, Torrado E, Saraiva M, et al. Pathological Role of Interleukin 17 in Mice Subjected to Repeated BCG Vaccination After Infection With Mycobacterium Tuberculosis. J Exp Med (2010) 207(8):1609–16. doi: 10.1084/jem.20100265
42. Wergeland I, Assmus J, Dyrhol-Riise AM. Cytokine Patterns in Tuberculosis Infection; IL-1ra, IL-2 and IP-10 Differentiate Borderline QuantiFERON-TB Samples From Uninfected Controls. PloS One (2016) 11(9):e0163848. doi: 10.1371/journal.pone.0163848
43. La Manna MP, Orlando V, Li Donni P, Sireci G, Di Carlo P, Cascio A, et al. Identification of Plasma Biomarkers for Discrimination Between Tuberculosis Infection/Disease and Pulmonary non Tuberculosis Disease. PloS One (2018) 13(3):e0192664. doi: 10.1371/journal.pone.0192664
Keywords: Mtb infection, protein signature, active TB, preventive therapy, soluble protein markers, cytokine and chemokines
Citation: Sivakumaran D, Jenum S, Ritz C, Vaz M, Doherty TM and Grewal HMS (2022) Improving Assignments for Therapeutic and Prophylactic Treatment Within TB Households. A Potential for Immuno-Diagnosis? Front. Immunol. 13:801616. doi: 10.3389/fimmu.2022.801616
Received: 25 October 2021; Accepted: 21 February 2022;
Published: 17 March 2022.
Edited by:
Novel N. Chegou, Stellenbosch University, South AfricaReviewed by:
Delia Goletti, National Institute for Infectious Diseases Lazzaro Spallanzani (IRCCS), ItalyNorman Nausch, Deutsche Gesellschaft für Internationale Zusammenarbeit, Germany
Copyright © 2022 Sivakumaran, Jenum, Ritz, Vaz, Doherty and Grewal. This is an open-access article distributed under the terms of the Creative Commons Attribution License (CC BY). The use, distribution or reproduction in other forums is permitted, provided the original author(s) and the copyright owner(s) are credited and that the original publication in this journal is cited, in accordance with accepted academic practice. No use, distribution or reproduction is permitted which does not comply with these terms.
*Correspondence: Harleen M. S. Grewal, Harleen.Grewal@uib.no; Synne Jenum, synjen@ous-hf.no